Introduction
Argon studies of hydrous K-bearing minerals on planetary surfaces
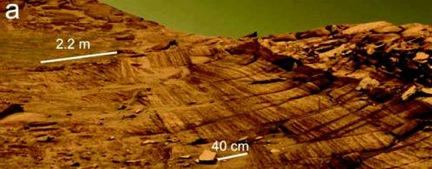
Jarosite, a potassium (K) bearing sulphate, was discovered within Meridiani Planum sediments by the Opportunity exploration rover using a Mossbauer spectrometer (Klingelhofer et al., 2004). Based on its occurrence on Earth, the presence of jarosite is an indicator of arid, acidic conditions following formation in a wet, acidic, and oxidizing environment (Fig. 2) (Elwood Madden et al., 2004). As a K-bearing mineral jarosite can be dated using the 40Ar/39Ar method (e.g. Vasconcelos et al., 1994; Lueth et al., 2005). Therefore, if jarosite-bearing samples were returned to Earth by future sample return missions to Mars, it is possible that the timing and rates of aqueous mineralization- and thus the time since an aqueous environment persisted on the Martian surface- could be determined by isotopically dating these minerals.
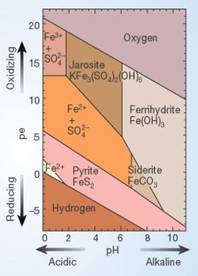
In preparation for Mars sample return missions, we are investigating the argon retention properties of terrestrial jarosites. Several samples have been provided by the Smithsonian Institution and others will be collected based on the applicability of their formation processes to those hypothesized for jarosite on Mars. The jarosite samples we have currently come from the Santa Eulalia district of Chihuahua, Mexico, the Mammoth Mine in the Tintic district in Utah, the Skouriotissa Mine in Cyprus, and the Meadow Valley mine in Pioche, Nevada. These jarosite samples have been characterized chemically by electron microprobe prior to performing argon diffusion experiments. Of importance is the abundance of potassium (K) in the samples (as wt% K2O), and the occurrence of chemically heterogeneous growth zoning (Fig. 3). Knowing the amount of potassium in the sample (along with a rough estimate for the age of the mineral) allows for optimization of sample irradiation parameters. Knowledge of high and low-K zoning within a sample provides insights into interpreting complex age spectra.
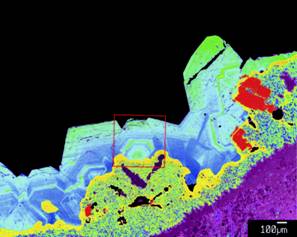
Following chemical analyses, mineral separates are prepared for irradiation and vacuum furnace step-heating in the Syracuse University Noble Gas Isotope Research Laboratory (SUNGIRL). Results of Ar diffusion experiments can be found here (Kula & Baldwin, 2011). Based on these results, more detailed degassing experiments will be designed that investigate the diffusion of argon in jarosite over a range of pressure-temperature conditions.
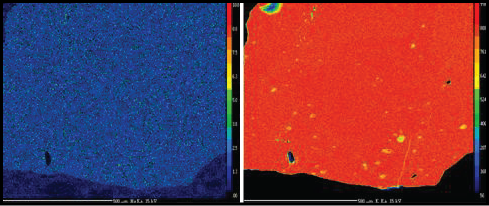
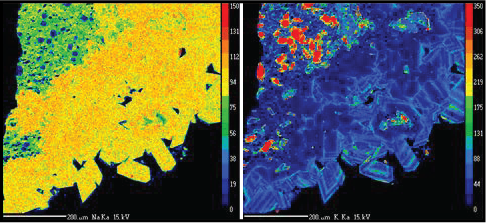
Argon diffusion experiments on lunar impact glasses and lunar-analogue compositions
The goal of this project is to answer the question: “Does Ar remain trapped in lunar impact glasses over geologic time or does it slowly diffuse out of the glasses?” The answer to this question has major significance with respect to efforts over the past decade to date lunar impact glasses via the 40Ar/39Ar method. If Ar diffuses out of these glasses over the billions of years while potentially exposed on the lunar surface this will cause the ages reported from 40Ar/39Ar chronology to be younger than the impact event that formed them.
Over the past decade 40Ar/39Ar ages on impact material have been used to test hypotheses relating to the timing and existence of the late heavy bombardment, and to assess if impact frequency can be related to mass extinction events on Earth. Understanding if these 40Ar/39Ar ages are accurately recording the timing of impact events has major significance for interpreting these ages in the context of the solar system’s evolution.
We will build on our collaborators’ previous work (e.g., Zellner et al. 2002; Delano et al. 2007) that used 40Ar/39Ar dating of lunar impact glasses to investigate the bombardment history of the Earth/Moon system. Both lunar glasses collected by the Apollo missions, and synthesized lunar glass analogue material with chemical compositions similar to lunar impact glass material, will be used for argon diffusion studies. Apollo lunar glasses (1-1.5 mm in diameter) will be characterized by electron microprobe and micro-Raman spectroscopy and subsequently irradiated in a nuclear reactor. The diffusion systematics of argon in lunar impact glasses will be determined as a function of chemical composition, and results will be interpreted with respect to the proposed impact history of the Earth-Moon system.
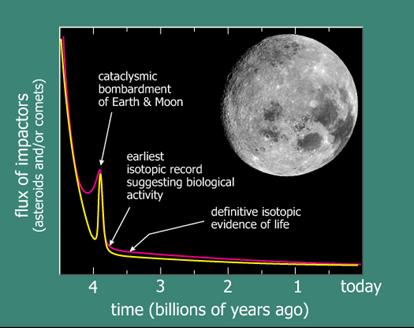
Ar diffusion experiments on plagioclase feldspar
Feldspars have been inferred to exist (5–15%) in the sedimentary rocks at Meridiani Planum and are known to exist in igneous meteorites from Mars. We plan to test the strategy of using feldspars in martian regolith (e.g., basaltic eolian sands) to infer local/regional/global history of volcanism (and thereby episodes of atmosphere additions and resulting climate change). Argon diffusion experiments in plagioclase are planned to assess kinetic parameters. These will be used to interpret plagioclase 40Ar/39Ar age spectra. Results will allow assessment of whether terrestrial volcanic histories can be extracted from plagioclase detritus in eolian sands from well characterized protoliths which in turn will be used in developing sampling strategies for a sample return mission from Mars.
References
Burger, P.V., Papike, J.J., Shearer, C.K., and Karner, J.M., 2009, Jarosite growth zoning as a recorder of fluid evolution: Geochimica et Cosmochimica Act, v. 73, p. 3248-3259.
Catling, D.C., 2004, On Earth, as it is on Mars?: Nature, v. 429. p. 707-708.
Delano, J.W., Zellner, N.E.B., Barra, F., Olson, E., Swindle, T.D., Tibbetts, N.J., & Whittet, D.C.B. 2007, An integrated approach to understanding Apollo 16 impact glasses: Chemistry, isotopes and shape, Meteoritics Planetary Sci., 42, 993–1004
Elwood Madden, M.E., Bodnar, R.J., Rimstidt, J.D., 2004, Jarosite as an indicator of water-limited chemical weathering on Mars: Nature, v. 431, p. 821-823.
Grotzinger, J.P., Arvidson, R.E., Bell III, J.F., et al., 2005, Stratigraphy and sedimentology of a dry to wet eolian depositional system, Burns formation, Meridiani Planum, Mars: Earth and Planetary Science Letters, v. 240, p. 11-72.
Klingelhofer, G., Morris, R.V., Bernhardt, B., Schroder, C., Rodionov, D.S., de Souza Jr., P.A., Yen, A., Gellert, R., evlanov, E.N., Zubkov, B., Foh, J., Bonnes, U., Kankeleit, E., Gutlich, P., Ming, D.W., Renz, F., Wdowiak, T., Squyres, S.W., Arvidson, R.E., 2004, Jarosite and hematite at Meridiani Planum from Opportunity’s Mossbauer spectrometer: Science, v. 306, p. 1740-1745.
Lueth, V.W., Rye, R.O., and Peters, L., 2005, “Sour gas” hydrothermal jarosite: ancient to modern acid-sulfate mineralization in the southern Rio Grande Rift: Chemical Geology, v. 215, p. 339-360.
McDougall, I. and Harrison, T.M., 1999, Geochronology and Thermochronology by the 40 Ar/ 39 Ar Method, 2nd edition, Oxford University Press, New York, NY, 269 p.
Vasconcelos, P.M., Brimhall, G.H., Becker, T.A., and Renne, P.R., 1994, 40Ar/39Ar analysis of supergene jarosite and alunite: Implications to the paleoweathering history of the western USA and West Africa: Geochimica et Cosmochimica Acta, v. 58, p. 401-420.
Zellner, N.E.B., Spudis, P.D., Delano, J.W., & Whittet, D.C.B. 2002, Impact glasses from the Apol lo 14 landing site and implications for regional geology, J. Geophys. Res., 107, 1–13